ElectrOasis integrates technologies for the growing of food, the collection of water, and the creation of electrical power for lighting and Internet connectivity. In 2015 Scientific American identified energy, food, and water as the world’s most critical resources. As such, ElectrOasis combines concentrated solar for energy generation, aquaponic fish farming symbiotically intertwined with producing organic vegetables, and water collection and green waste management. ElectrOasis conflates available technologies into integrated artificial and natural ecosystems that are scalable, sustainable, and designed for desert or urban landscapes in arid or wet regions of our planet.
ElectrOasis is designed to harvest sunlight and available discarded natural resources such as paper and green waste to create energy, food, communications hubs, and places for researchers and scholars to connect to the web. An oasis is a special place in the desert where life-giving water and shade can be found, though it is also a fertile patch in a desert occurring where the water table approaches or reaches the ground surface. An oasis is also a place of peace, safety, and happiness, sometimes amid trouble or difficulty.
This ElectrOasis will provide shade, freshwater, and plant life. ElectrOasis will evolve and extend the oasis notion by constructing a place where water will be collected from moist air, and fresh fish will be grown to fertilize edible vegetables. Power generation from daylight solar driving a small turbine will allow internet connectivity and the creation of interior lighting to empower reading, knowledge generation, and peace.
It will be where information and web connectivity will allow locals and visitors to have connectivity and agency in a world, especially for educational experiences surrounding green technologies.
This ElectrOasis project empowers all to use what is at hand and conflates high technologies and ancient wisdom in new ways. It allows common materials, like pipes, sand, car parts (generators), solar collectors, water, tilapia fish, and green waste (paper, vegetable, meat discards, and animal waste), in an integrative and systemic way.
These integrated systems can function in deserts where sunlight and water are scarce in urban environments. It can also be adapted for any region where people are abundant with massive needs, though materials and infrastructure are lacking.
Growing Fish: Aquaponics
For over 5000 years, the Egyptians were known for their aquaponic farming, and for thousands of years, tilapia were grown in fish farms in the Nile delta.
With ElectrOasis we will re-introduce tilapia farming as a primary source of creating protein for human consumption and relaxed, peaceful viewing.
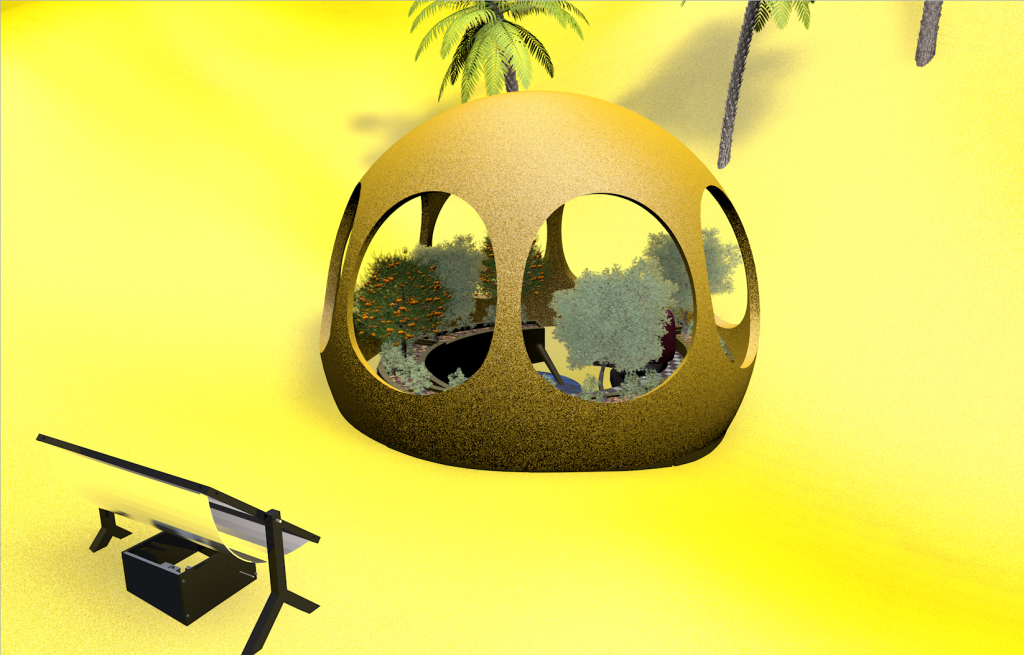
Nile Tilapia are distinctive with regular, vertical stripes extending as far down the body as the bottom edge of the caudal fin, with variable coloration. Adults reach up to 60 cm (24 in) in length and up to 4.3 kg (9.5 lb.). Tilapia live for up to 9 years and can tolerate brackish water, and survive in temperatures between 8 – 42 °C (46 – 108 °F). Tilapias are omnivorous and feed on plankton as well as on higher plants. In recent research done in Kenya, this fish has been shown to feed on mosquito larvae, making it a possible tool in the fight against malaria in Africa, though; for this project, we are going to create a black soldier fly farm to automatically feed the tilapia.
I have successfully implemented aquaponics systems in three past projects in collaboration with Amy Youngs.
Two were indoor systems in urban environments, and one was in a desert region of Portugal.
Please refer to the Farm Fountain and Solar Farm Fountain. The Farm Fountain won a Green Leaf Award from the United Nations Office of Environment in 2008.
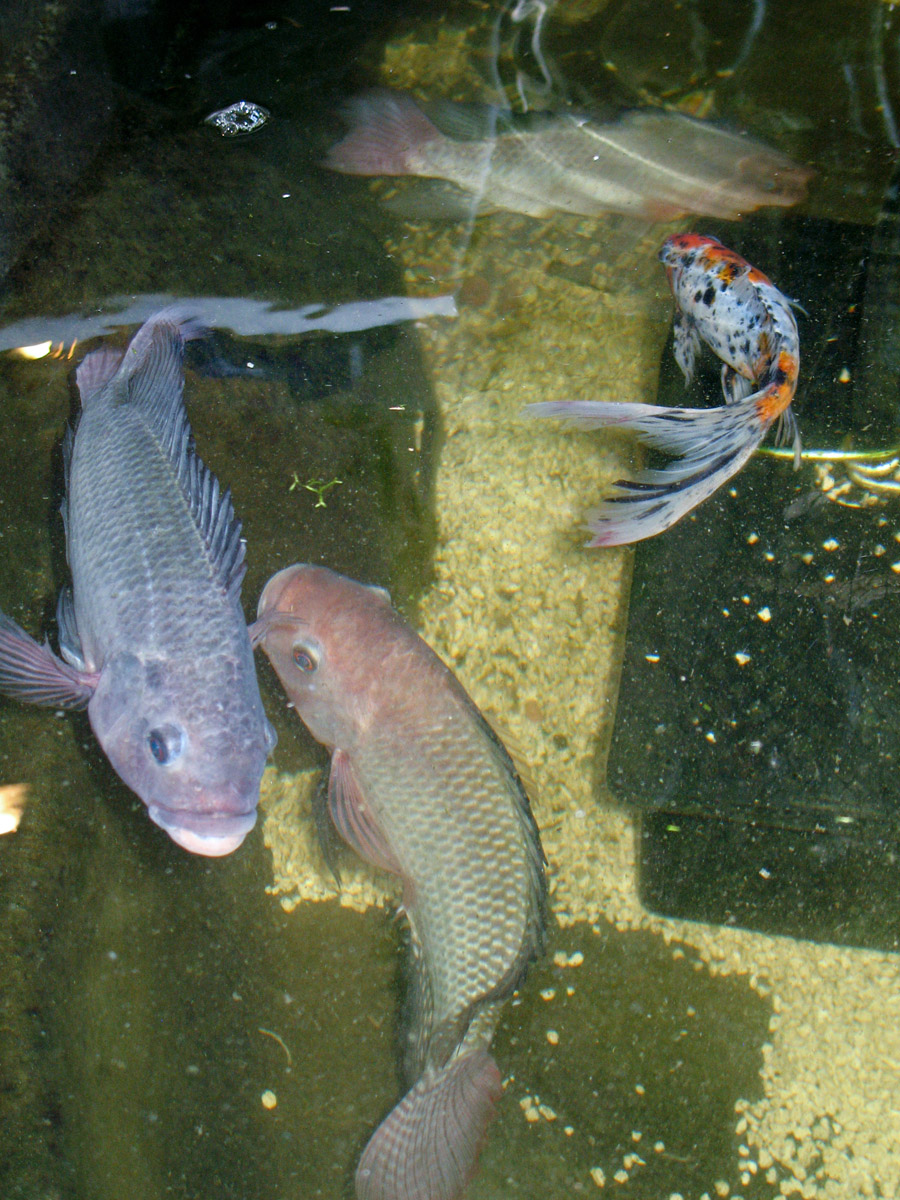
The tilapia will reside inside the tank below the dome in this installation. The tank will be 300-500 gallons giving the ability to grow 230 Tilapia at one time. 230 tilapia can create quite a bit of fish waste. The great thing is that fish waste makes some of the best fertilizers on the planet when combined with nitrifying and denitrifying bacteria. All the fish waste from this system will be pumped into cleansing beds filled with plants and bacteria, and this will serve to cleanse and return the water to the tilapia tank.
Growing Organic Vegetables: Food
Flow-through systems will be implemented around the periphery of the dome on the interior to maximize available fish and bacterial nutrients by orienting the grow beds so that pumping water to one height will effectively water all plants.
Plants will be chosen to provide maximum growth given the region’s sun cycles and primary crops. Areas for shade and areas for more sun will be implemented using screening materials on the interior of the dome.
Microprocessors will be used to monitor water/soil PH and allow varying amounts of fertilizing inputs or not, given the plant’s needs.
Typical recipes rely on the herbs; parsley, cilantro, mint, basil, rosemary, oregano, bay leaves, and thyme, and spices like garlic, anise, saffron, cinnamon, caraway, coriander, cumin, fennel, fenugreek, ginger, white pepper, black pepper, red pepper, and cloves will be tested in the system. Vegetables such as onions, bell peppers, carrots, chickpeas, tomatoes, capers, celery, turnips, potatoes, chili peppers, cucumbers, and eggplants will be explored as suitable for the growth beds.
The benefit for the human visitors will be the sound of cleansed water trickling as it reenters the main tank, creating a relaxing, focused aspect of experiencing the space.
Growing Power: Energy/Knowledge
For some time, concentrated solar has been an effective method of generating power in desert regions. With the generation of steam to drive turbines, this proposed system will allow a low-cost/maintenance solution and entrance into power generation without massive cash outlay.
NUR Energy has been working with concentrated solar with their most recent large-scale installations in Tunisia. They have developed the world’s first CSP solar export project between North Africa and Europe. It is located in the Sahara desert and has a solar radiation level of 2500 kWh/m2 annually.
Sill, concentrated solar can be achieved with materials such as low-tech mylar sheets pulled into parabolic solar lens concentrators with vacuums layered into the garbage can tops and then creating a vacuum to create the parabolic. What is essential here is the knowledge… and ElectrOasis will be the repository for that knowledge, for how to grow food and excel, given the natural resources adequately funneled through advanced technologies and methodologies to successfully implement these integrated systems.
For this proposal, we have selected a solar concentrator manufactured out of polished aluminum for durability.
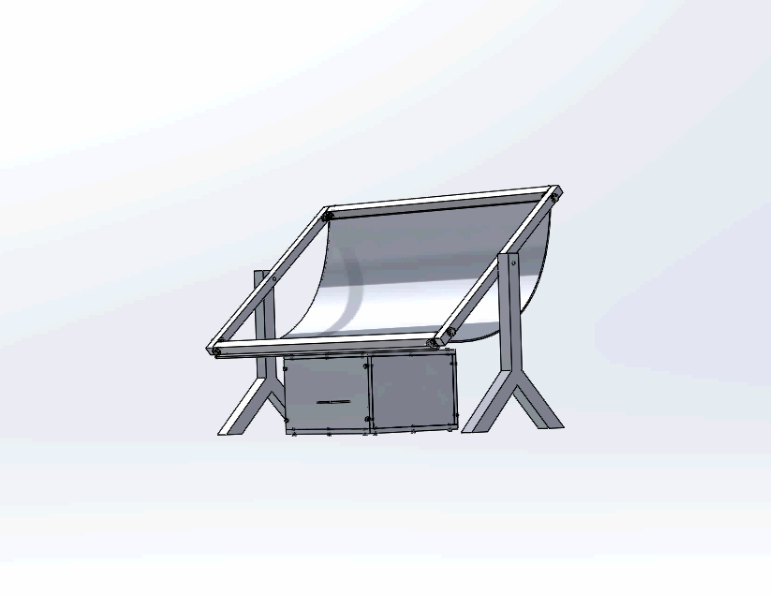
What is important here is that the solutions and integration of these technologies are scalable. More or less advanced manifestations of the ideas are possible, given the desire for a generation of power, water collection, and food growing.
What will we do with that solar?
We will use standard pipes at the apex of the solar concentrator to create steam. The steam will drive a revolutionary turbine called the TESLA Turbine, which will be attached to a standard car alternator to generate power. The pipes that distribute this steam will be standard pipes used for cars, such as brake lining pipes designed for extreme temperatures and pressures for safety.
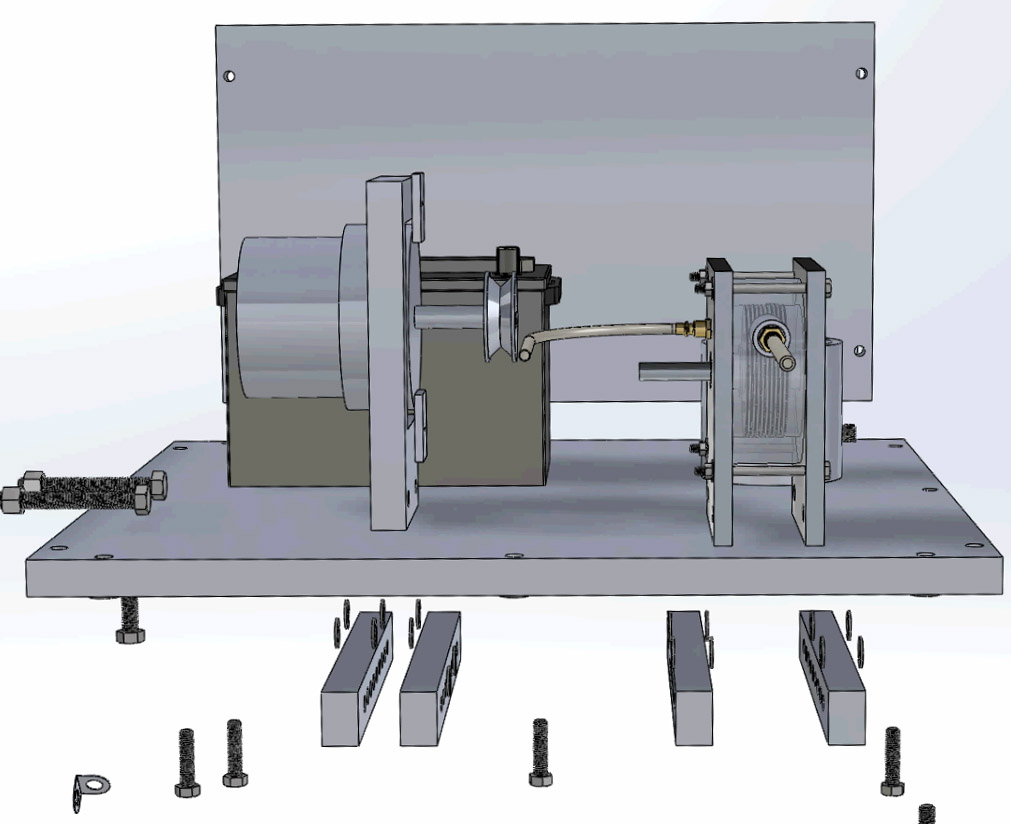
Deep-cycle marine batteries (power storage) with existing power generators (car generators) are available in any country. They will allow the system to provide light and water pumping during the hours that the sun is not shining.

Where existing solar cell technologies are affordable and available, these methods are also possible; i.e., using solar concentrators also shining on solar cells. High-tech and efficient LED technologies will be implemented inside the dome and focused on the seating area to create light in the evening for reading and learning about green technologies.
Part of this project will be to create a web page devoted to green energy solutions in water collection, production of steam, ways of using the steam to generate power, and methods of growing food using organic and recycling means such as aquaponics and vermiponics. This can be an ongoing open-source project seeking local community input and connecting to worldwide web resources. Any desert region or place with adequate sunlight can be a possible site for the project with unique flora, fauna, and cultural landscape knowledge that will be distilled and propagated as to best practices given local environmental factors.
Another option to get water to flow uphill is the ram pump as it is simple and able to pump water uphill.
Recycling Waste/Feeding Fish: Vermiponics and Black Soldier Flies
Using controlled methods of introducing green waste, worms would be fed paper and food scraps to allow for the production of soil and plant fertilizers. These systems will be integrated with the river as a model, where water that comes directly from the waste of fish would be pumped into gravity-fed systems starting with marsh-like conditions where fish solid waste would be denitrified by bacterial cultures, as it progressively flows through cleaner and cleaner containers also feeding organic vegetables.
The fish waste is turned into the best fertilizer the plants would want to consume and, in the process, sets up a symbiotic space where fish, plants, bacteria, worms, and humans benefit from each other.
An additional technology to be implemented will be black soldier fly harvesting zones. These are beneficial as the grubs the black soldier flies birth is the ideal food for tilapia. Black soldier flies also consume more food waste than red wiggler worms.
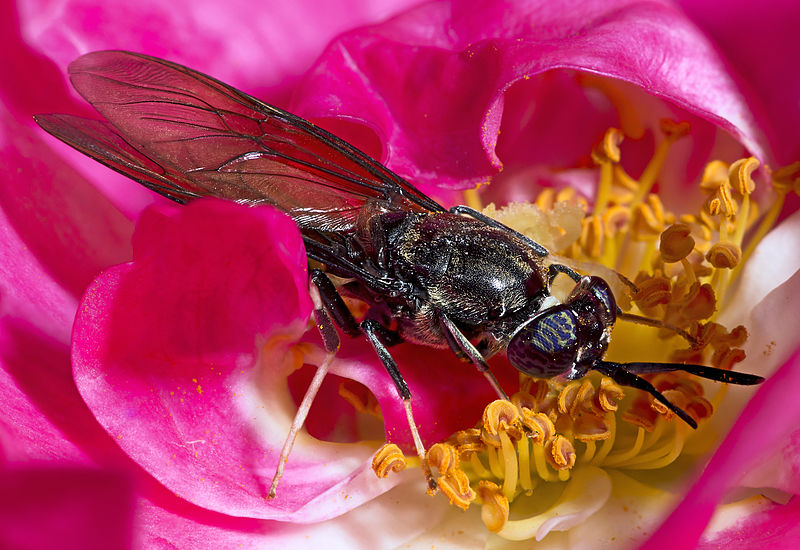
While the thought of flies may seem gross, they are the ideal solution to not overfishing our oceans (normal food for aquaponic farms). They don’t carry diseases, they cannot bite, or sting and experts report their voracious appetites for eating compost scraps (including pet waste) and eliminating most food scraps in 24 – 36 hours.
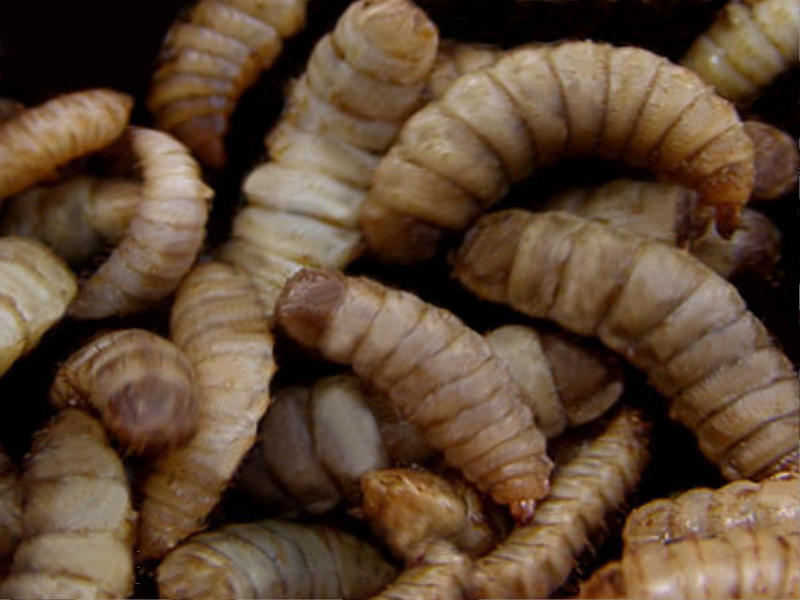
They also prevent houseflies and blowflies from laying eggs in the material inhabited by black soldier fly larvae. The fantastic thing about the soldier fly larva is that once they are born, they have an innate ability to crawl up an incline of 35 degrees, and this will be exploited in the design where a gradient is created in the waste reprocessing boxes and that waste stream, will have a direct pipe feeding into the tilapia tank. This system empowers biology to do what it does best.
One of the challenges of growing food in a desert is the creation of fertile soil. New land doesn’t have organic matter, so you must build it up with compost, and the worms and soldier flies will be part of that natural process.
Efficient evaporative coolers integrated into the system will maximize the creation of mini Oases for the worms, the flies, and the fish.
The UNC Institute for the environment http://www.ie.unc.edu/for_students/courses/capstone/13/bsfl_how-to_guide.pdf “Temperature The optimal temperature at which BSFL consumes their food is around 95 °F. The minimum temperature for survival is 32 °F for no more than four hours, whereas the maximum temperature is 113 °F. The larvae become inactive at temperatures less than 50 °F and above 113 °F.
The best range of temperature for the larvae to pupate is from 77 to 86 °F. The optimal temperature for mating is around 82 °F (Zhang, 2010).
Diet BSFL can tolerate a widely varied diet. The BSFL feeds on many kinds of organic waste, such as table scraps, composting feed, and animal manure. They can also survive off of coffee grounds for a few weeks, but coffee grounds are not a sustainable diet. The caffeine from the coffee grounds helps to boost the metabolism and makes the grubs more active. A diet combining kitchen scraps and coffee grounds may help to boost their metabolism.
The BSFL has a limited ability to process animal products such as meat and fat. Humidity Black soldier fly larvae develop most rapidly at 70 percent humidity.
The rate of weight loss for the BSFL increases with decreasing humidity. The optimal humidity for black soldier fly mating is around 30 to 90 percent.
It is very important to monitor humidity for captive rearing and breeding. We found that it is especially important to keep the grubs’ feeding medium at a proper moisture level—not so dry that it cements the grubs into the feed and not so wet that they cannot breathe through the pores in their exoskeleton. In additional environmental conditions, BSFL does not survive well in direct light or extremely dry or wet conditions. They prefer to be 8- 9 inches deep in their food source. They will perform little bioconversion if they are too far below the surface. Female flies avoid anaerobic sites when trying to lay eggs.”
Another consideration is to use Nannochloropsis, which is a food additive for human nutrition and is also served at Restaurants. A 2020 study suggests it could be used for sustainable fish-free feed for farmed fish.[1]
Growing Thirst: Water Collection
Using the biomimetic design, I have also designed water collecting mesh systems into the dome that allows collecting water from fog in the desert. The Carapace and Namib beetles, native to the Namib desert of southern Africa, are known to pull water from the air. We will use multiple layers of stainless steel mesh that roll around the dome structure in the evenings to collect the moisture in the philosophy common in this region of Tunisia, to collect and funnel that back into the main tilapia fish tank as the curtains will hang above the filtration tanks and fall into the cleansing stream.
The system’s efficacy will depend on the size of the filaments in the nets, the size of the holes between the filaments, and the coating applied to the filaments.
Research done at MIT indicates stainless-steel filaments about three or four times the thickness of a human hair and spaced at twice the distance between fibers are optimal.
Further research indicates a yield of a few liters of drinking water per day for each square meter of mesh deployed. As curtains on the structure are possible and given the openings in the system, we would estimate we can collect 6-12 liters of water per evening in this fashion. These screens can be deployed in the evenings for fog collection of water or in the day for more shade when temperatures hit extremes.
We will use inexpensive Arduino Microcontrollers in parallel to monitor many subsystems, such as water pumping with solid-state relays, maintaining interior temperatures with sensors, and controlling high-efficiency LED lighting for evening illumination.
Growing Structures: Architecture
Research into traditional Tunisian architecture determines the methods of building the structures to house the solar collection systems, aquaponics, vermiponics, and veggie growing beds. Vaulted adobe buildings reinforced with concrete will create covered areas for fish and plants with semi-shade.
One possible method to build the dome would be to use mounded earth moistened with water, covered with plastic, and make the structure on top of the mound. Once the adobe or reinforced concrete is cured, the mound is removed, leaving the dome structure shell.
There are many methods of building the structure, and another one that could be employed is the earth-bag, where bags function as large coil pots that progressively wind around the system. http://earthbagbuilding.com/projects/thailand.htm
An additional method that may be explored is using plastic bottles to create plastic bottle structures. This method has been successfully used worldwide where building materials are scarce though waste plastic bottles are plentiful. The technique is labor-intensive and would require many participants to allow it to happen.
A rounded seating area will allow locals to sit and watch the fish, monitor the system, provide shade, and a place for reading at night and connecting to the web for further research and political agency in learning. In parts of the world where building materials are scarce, existing structures can house aquaponics and plant-growing areas. Concrete recycling can also be explored as a means of getting materials that are readily at hand from destroyed buildings.
For this project, the team focused on developing a robust power generation with the TESLA Turbine. However, the Tesla turbine is not the only way to power this system. Solar cells with solar concentrators would also be suitable. Tesla turbines have been built with high-tech laser cutting or as low-tech as recycling metal hard disks from discarded computers.
Methodology
Professor Rinaldo worked with engineering interns who were able to actualize his designs. This turbine was tested with compressed air, though it will be powered by steam generated by linear solar concentrators.
The optimal turbine design lies in synthesizing efficiency, reliability, and feasibility. The team performs preliminary design analysis to establish the plan based on efficiency computationally. Previous experimental works have determined factors such as distance between the micro-turbine disks, number, and diameter of the micro-turbine disks, the number of inlet nozzles to the micro-turbine, and rotational speed of the rotor affect the efficiency of the turbine [2]. In particular, we will be concentrating on measuring isentropic efficiency. This parameter measures the degree of degradation of energy in a steady-flow device. It involves comparing the actual performance of the device and the performance that would be achieved under idealized circumstances for the same inlet and exit states. [3]
The team aims to explore how these mechanical design considerations can be adapted to ensure long-term reliability while employing readily available, cost-efficient materials. This will aid in our goal of constructing the turbine in a manner that caters to future production.
In similar systems, heat transfer fluids such as oils or molten salts are heated in linear troughs. These fluids then boil water that flows in a conventional steam-turbine generator [4]. Our team intends to explore the possibility of solar concentration allowing for significant design simplification. I believe these modifications are a better method of powering a turbine for low-power applications as they have few moving parts and can be manufactured more quickly. First, it is less costly due to fewer required storage and heating tanks. Furthermore, the modifications allow the team to employ an elementary energy storage system attuned to low-power applications consisting of a car alternator and deep-cycle car battery, making this system accessible to poor communities.
Power production is the ultimate goal of this project, and thus the discovery of the maximum output wattage is critical. For this, turbine testing can follow in the footsteps of the experiments conducted by Vincent Domenic Romanin in his dissertation Theory and Performance of Tesla Turbines though this is not yet complete. Output power, battery capacity, and the power requirement of the electrical consumers must be matched to ensure sufficient current is supplied to the electrical system in all operating conditions and that the battery is always adequately charged [5]. This will be primarily answered during the validation of our design.
Creation of Specifications and Requirements
The ElectrOasis team went through an intense preliminary design phase to bridge the concept to CAD design gap. The early project configurations were conceptualized and defined as schematics, diagrams, and layouts of the project were completed. Specifications were chosen based on materials that will be robust and readily available to others wishing to replicate this design. Requirements include but are not limited to: the cost of purchase and repair of the turbine, size and overall dimensions of the power systems housing, ease of operation and repair, and reliability of the turbine.
Creation of Design Concepts
The team has worked over the past two weeks to produce a complete working drawing set of the design concept in SolidWorks. First individual parts were created with careful consideration of future construction difficulties. The details were toleranced with attention to the particular fit (i.e., clearance fit, transition fit, or interference fit). Next, the elements were combined into an assembly to understand how they function together. Examples from the drawing set can be found below in Figure 3.
The team has met with mechanical engineering professor Prasad Mokashi to investigate computational fluid dynamic analysis in ANSYS. This simulation software will allow the team to predict, with confidence, the impact of fluid flows on the turbine—throughout design and manufacturing as well as during end-use.
Solution Design/Creation of Prototype
This design phase consisted of the fabrication of the turbine. The team will send the parts to Allfab Incorporated for advanced fabrication processes to be machined. This design phase will show the group how the proposed solution will function in “real life” and how it interacts with real-world environmental constraints. It will provide the basis for future testing and will be representative of performance.
Testing/Validation of Design
In the final stage of the process, the team will evaluate the performance of the created turbine. We will be able to get an actual power estimate and extrapolate that to predict what the system will be capable of powering once implemented in Tunisia.
Testing will occur in three primary stages. First, we will conduct compressed air tests to calculate torque and RPM values as air pressure functions. This testing will be independent of the solar concentration and indicate the turbine’s performance. Next, the team will conduct prolonged steam testing to evaluate long-term concerns such as warping in the turbine. Finally, steam testing with the alternator and battery will ensure the system is closed and gauge the net power output.
Qualifications
The power systems production team comprises Professor Ken Rinaldo overseeing Colin Trussa, Anthony Huang, and Yizhou Lu. As Mechanical and Aerospace engineering students, they/we have access to extensive research databases, laboratory equipment, and modeling software. Our combined skill-sets and backgrounds provided an excellent basis for this project’s mechanical design and implementation and the TESLA Turbine in particular. Our team consists of three other essential members, Associate Professor Amy Youngs (Art: Art & Technology), an expert in aquaponics and vermiponic Gardening, and myself (Bio-artist, robotics art) working with Jeremy Viny, an Art student studying green technologies, and Monica Backs Environmental Engineering student, researching plants.
Additional plant Research:
General information:
http://www.nationsencyclopedia.com/Africa/Tunisia-AGRICULTURE.html
Olives, grapes, wheat, barley, peppers, dates, peppers, and potatoes are the primary produce grown in Northern Tunisia. Since we are trying to grow the plants indoors, with a system that will allow us to keep the soil moderately moist, I believe we will have a wide range of plants to choose from.
Barley:
http://www.aglime.org.uk/tech/crop_requirements.php
Barley is excellent at preventing soil erosion, suppressing weeds, “scavenging” for excess nutrients, and adding organic matter to the soil. Barley is also widely mixed with other crops because it can serve as topsoil and protect the plants with which it grows during drought. Barley doesn’t grow well in waterlogged soil and seems to do very well on light, droughty earth with a higher sand and clay content. Its roots can grow to be up to 6.5 feet deep. Barley prefers soil with a pH range from 6.5-7.5.
Pros:
- Intercropping barley increases the amount of N absorbed by barley and returned to the soil in barley residue. It also shows increased amounts of P and K recycling.
- It can grow in drier climates.
- Can protect other plants within the ElectrOasis.
Cons:
- Establishes deep root systems- do we have the room?
- Usually planted in large quantities/ requires a large surface area.
Potatoes:
https://www.sproutabl.com/gardening/vegetables/potatoes/
http://www.almanac.com/plant/potatoes
http://www.bettervegetablegardening.com/fertilizing-potatoes.html
Potatoes grow better in cooler climates and out of direct sunlight. They thrive in well-drained but consistently moist soil. Potatoes plants prefer acidic soil and can be damaged by “Potato Scab” when soil pH is too basic. The maximum growing pH is 6.5, with careful observation to prevent Potato Scab.
Pros:
- Enjoy constant moisture that could easily be provided within the ElectrOasis.
- Enjoy nitrogen-rich soil, and usually not at the expense of the vegetables.
- Relatively short roots (~24 inches)
Cons:
- Recommended to plant them 1 foot apart.
- Require “hilling” as they mature to prevent sunburn.
- It is advised to practice “crop rotation,” meaning they could not constantly be replanted within the ElectrOasis.
Tomatoes:
http://www.almanac.com/plant/tomatoes
Tomatoes prefer at least 6 hours of sunlight per day as they grow. Well-drained soil is necessary for the success of a tomato plant. Tomatoes prefer loamy soil with a more acidic pH.
Pros:
- Thrives in acidic soil, similar to potatoes.
- Healthy plants produce ample vegetation.
- Root systems can be fairly shallow (~18 inches).
Cons:
- They need to be staked as they grow to support the weight of the plant.
- Require a decent amount of sunlight.
- Too much nitrogen within the soil can cause the plant to grow much larger at the expense of the fruit (large plant with no tomatoes).
Bell Peppers:
http://www.almanac.com/plant/bell-peppers
Bell peppers prefer neutral soil and full exposure to the sun. They grow best in warm environments (+70 degrees F). These plants prefer moist but well-drained soil.
Pros:
- Grow best in warm environments.
- Vegetable contains a significant Vitamin C content.
Cons:
- They need to be staked as they grow to support the weight of the plant.
- Require direct exposure to sunlight.
- Too much nitrogen within the soil can cause the plant to grow much more significantly at the expense of the fruit (large plant with no peppers).
The most successful path for water purification with these four plants would be potatoes, tomatoes, and bell peppers.
http://www.conservationevidence.com/actions/719
It is proven that potatoes have a higher yield while rotated with Barley… Is it possible that we could make it work? Regardless, most of the vegetation used in Tunisian cooking requires much attention during their youth.
Spices and Herbs:
Parsley:
http://www.almanac.com/plant/parsley
Pros:
- High in Vitamins C and A.
- Prefers soil at a temperature of 70 degrees F or higher.
- Proven to have symbiotic growth with tomatoes. Plant this near tomato!
- Loamy soil.
Cons:
- Requires even watering and often.
- Grow best in rich soil full of organic matter; this may be an issue for the plants as the ElectrOasis is in its early stages.
Mint:
http://www.almanac.com/plant/mint
Pros:
- It grows well near tomatoes!
- Aromatic, calming smell.
- Minimal care is usually required.
- Loamy soil.
Cons:
- It can take over an entire garden due to the spreading root system.
- Requires even watering.
Basil:
http://www.almanac.com/plant/basil
Pros:
- Loamy soil.
- Easy to grow; it requires soil above 70 degrees F.
- It grows well near tomatoes!
Cons:
- Need to be planted about 10 to 12 inches apart.
- Require constant moisture.
Thyme:
http://www.almanac.com/plant/thyme
Pros:
- Likes sandy to loamy soil.
- Has a pleasant fragrance.
- Small; it only grows 6-12 inches in height,
Cons:
- Difficult to grow.
- Requires a lot of pruning and attention.
https://en.wikipedia.org/wiki/Tunisian_cuisine#Ingredients
Typical Recipes rely on:
Herbs: parsley, cilantro, mint, basil, rosemary, oregano, bay leaves, and thyme.
Spices: garlic, anise, saffron, cinnamon, caraway, coriander, cumin, fennel, fenugreek, ginger, white pepper, black pepper, red pepper, and cloves.
Vegetables: onions, bell peppers, carrots, chickpeas, tomatoes, capers, celery, turnips, potatoes, chili peppers, cucumbers, and eggplants.
References
1.) Causes of Aridity and Geography of the World’s Deserts. Retrieved October 16, 2015
2.) Lampart, P., Kosowski, K., Piwowarski, M., & Jędrzejewski, Ł. (2009). Design analysis of Tesla micro-turbine operating on a low-boiling medium. Polish Maritime Research
3.) Huang, M., & Gramoll, K. (n.d.). Thermodynamics eBook. Retrieved October 18, 2015.
4.) Concentrating Solar Power (CSP) Technology. (n.d.). Retrieved October 16, 2015.
5.) Alternators. (2007). In Bosch automotive electrics and electronics: Systems and components, networking and hybrid drive (5th ed.). Plochingen: SpringerVieweg.
6.) Romanin, V. (2012). Theory and Performance of Tesla Turbines.
7.) Brownson, J. (2014). Solar energy conversion systems. Oxford: Academic Press.
8.) ENGR 1281.03H Lecture 37: Engineering Design Process. (2014). Retrieved October 18, 2015.
9.) DESERTEC Foundation: Technologies. (2015). Retrieved October 10, 2015.
10.) 2015 World Hunger and Poverty Facts and Statistics by WHES. (n.d.). Retrieved October 18, 2015.
11.) Chandler, D. (2012, August 6). Bringing power to the people – and heat as well. Retrieved October 18, 2015.